Key to harnessing light waves? First do the math
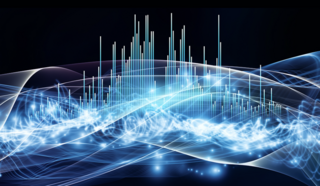
Hot bodies emit light waves in a process scientists call ‘thermal radiation’ – exemplified in the light we see through night vision goggles or in the glowing embers of a fire. New thermophotovoltaic devices capture these energy waves, offering the potential for more efficient and sustainable electricity than offered by conventional solar cells.
But until now scientists have been unable to measure the maximum rate at which thermal energy can be transferred from a hot body to a cold photovoltaic.
Scholars at Yale’s Energy Sciences Institute have for the first time developed a mathematical model that effectively predicts a maximum rate of thermal energy transfer. Published in Nature Communications, the new theory sidesteps the requirement for endless checking of every possible permutation, instead revealing upper limits to what is possible.
“We realized that there’s a different way of viewing wave scattering that’s mathematically analogous to how we interpret electrical circuit response, or scattering by atoms and molecules” said Owen Miller, an assistant professor of Applied Physics at Yale and primary author of the study.
First author Lang Zhang, a graduate student in the Miller group, has dedicated her studies to developing new ideas in radiative energy transfer. Last year, the team collaborated with scholars at the University of Michigan to experimentally demonstrate record-breaking “near-field” energy transfer.
Their new theoretical framework reveals an otherwise hidden structure in complex wave scattering.
As well as establishing crucial understanding for next gen energy-harvesting technologies, the framework is also expected to have an impact on wide-ranging challenges in optics and photonics, from isolators that protect laser systems to better designs for augmented-reality technologies.
The theory may also be useful in resolving open questions in other areas of wave scattering, including acoustic, fluidic, and quantum phenomena.
In addition to Miller and Zhang, Francesco Monticone of Cornell University collaborated on the study, which was funded by the Air Force Office of Scientific Research and by the Army Research Office under a MURI (Multidisciplinary University Research Initiatives) program.